Book contents
- Caplan’s Stroke
- Caplan’s Stroke
- Copyright page
- Contents
- Preface
- Contributors
- Part I General principles
- Part II Stroke syndromes
- Part III Prevention, complications, and recovery–rehabilitation
- Chapter 18 Stroke prevention
- Chapter 19 Complications in stroke patients
- Chapter 20 Recovery, rehabilitation, and repair
- Index
- Plate section
- References
Chapter 20 - Recovery, rehabilitation, and repair
from Part III - Prevention, complications, and recovery–rehabilitation
Published online by Cambridge University Press: 05 August 2016
- Caplan’s Stroke
- Caplan’s Stroke
- Copyright page
- Contents
- Preface
- Contributors
- Part I General principles
- Part II Stroke syndromes
- Part III Prevention, complications, and recovery–rehabilitation
- Chapter 18 Stroke prevention
- Chapter 19 Complications in stroke patients
- Chapter 20 Recovery, rehabilitation, and repair
- Index
- Plate section
- References
Summary
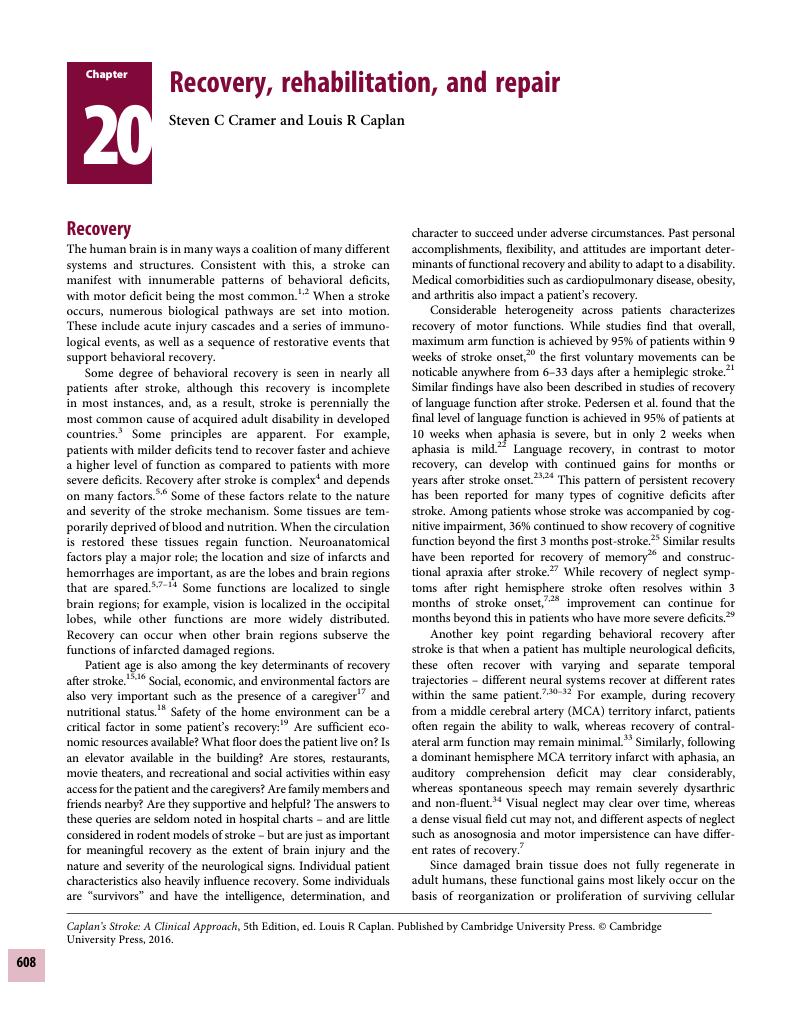
- Type
- Chapter
- Information
- Caplan's StrokeA Clinical Approach, pp. 608 - 626Publisher: Cambridge University PressPrint publication year: 2016